Creating a Powerhouse for Energy Solutions
Arts & Sciences researchers see collaboration as key to recharging the planet’s battery.
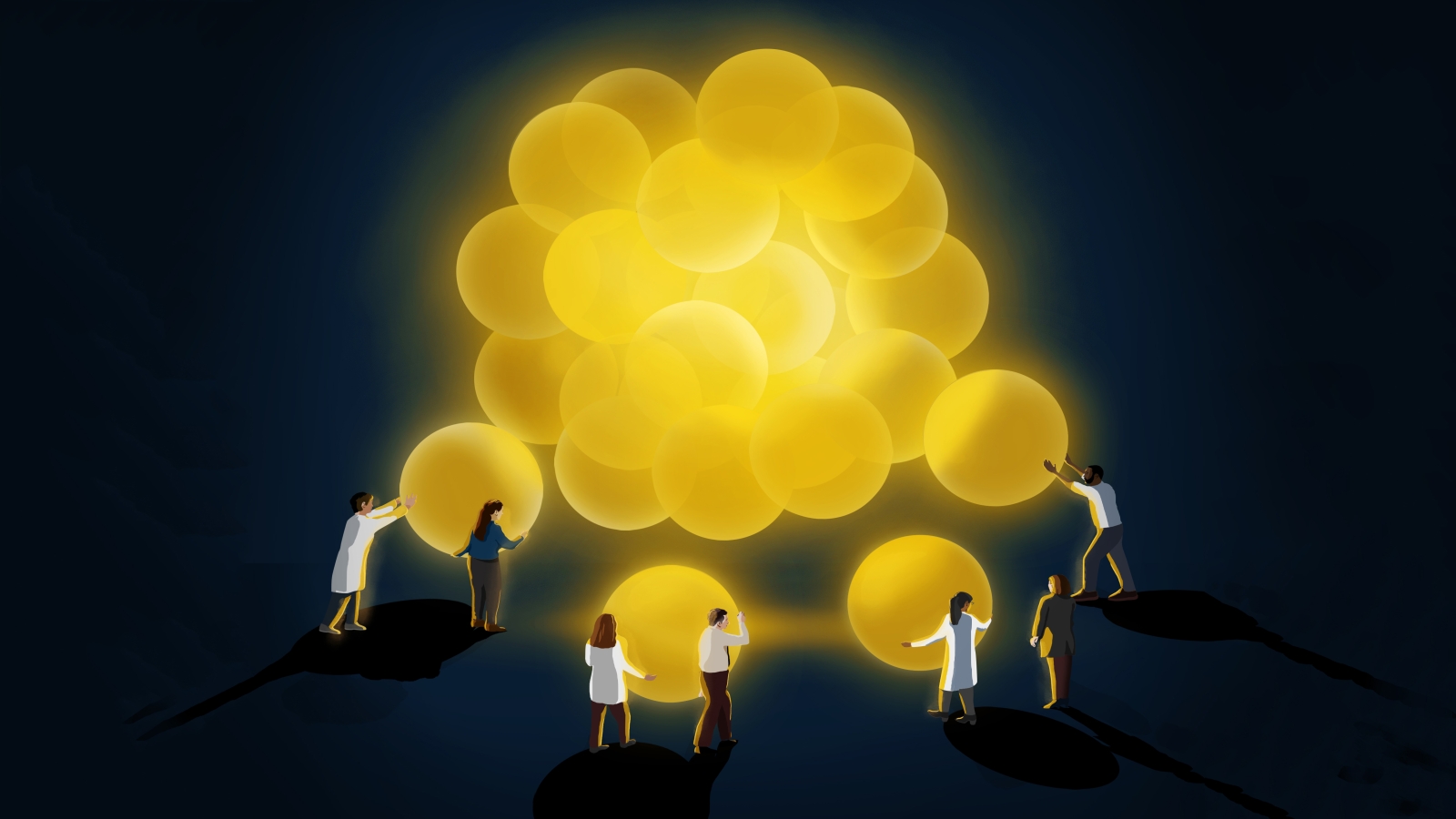
Non-renewable fossil fuels like coal, oil, and natural gas supplied 81 percent of the world’s energy in 2017. This level has remained stable for more than 30 years, even as the scientific community has developed an overwhelming consensus that human activity—including the harvesting and use of these fuels—is having a negative impact on the global environment.
“Our society has been dependent on fossil fuels for a long time, and there’s a lot of innovation necessary before society can fully transition to energy sources that are renewable and less harmful to life on earth,” says Karen Goldberg, Vagelos Professor in Energy Research in the Department of Chemistry. “The energy situation is one of the most serious issues facing us today. But it is vital that the solutions that we go after are economically viable and can work on an industrial scale. And along with the science, we are going to need policy changes to help us make the transition successful.”
Energy has been a focus for faculty and students throughout Penn Arts & Sciences and the rest of the university, as they put Penn’s practical approach and collaborative ethos into action with research and education. One example is the Vagelos Integrated Program in Energy Research (VIPER), in which undergraduate students earn dual degrees in science and engineering, conducting research and receiving close mentorship. “The students come to Penn wanting to make a difference,” says Andrew Rappe, Blanchard Professor of Chemistry and Faculty Co-Director of VIPER. “They realize this is going to continue to be a challenge for a long time and that it’s a challenge that needs them.”
Another response to the challenge is the creation of the Vagelos Institute for Energy Science and Technology, supported by a gift from P. Roy Vagelos, C’50, PAR’90, HON’99, and Diana T. Vagelos, PAR’90. As the hub for energy research at Penn, the Institute pulls together investigators to facilitate communication and cooperation, provides seed funds for new collaborative research projects, and is building connections to industry to put findings into action.
“Today we’re faced with the specter of climate change fundamentally altering not just local weather or coastlines or even economies, but the planet’s ability to sustain life,” says Steven J. Fluharty, Dean of Penn Arts & Sciences and Thomas S. Gates, Jr. Professor of Psychology, Pharmacology, and Neuroscience. “We have made addressing this threat a top priority of the School, and with the Vagelos Institute we are putting the pieces in place to make a tangible impact on this complex set of challenges.”
The catalyst for the Institute is Goldberg, who was recruited after an international search to be its inaugural director. She is a member of the National Academy of Sciences, and her groundbreaking work to develop new systems that can efficiently produce chemicals and fuels from materials like natural gas and carbon dioxide earned her the American Chemical Society’s Award for Organometallic Chemistry.
More than that, as the former director of a National Science Foundation Phase II Center for Chemical Innovation, the Center for Enabling New Technologies through Catalysis (CENTC), Goldberg has 10 years of experience in mobilizing collaboration among investigators and taking a big science approach to solving energy problems.
When Goldberg came to Penn she began holding weekly meetings with about 30 researchers from Penn Arts & Sciences and the School of Engineering and Applied Science. Her goal was to get the investigators in the same room to talk about their research, trends, and opportunities to collaborate.
“Hearing about what other people are working on is important because it sensitizes you to the challenges in a variety of fields around energy,” says Eric Schelter, Professor of Chemistry. “We can then think about how our group can bring our capabilities to bear on these challenging problems.”
At these meetings, scientists and engineers worked together to identify an initial set of priority research areas they saw as having a big potential impact and in which the expertise at Penn could best be combined in synergistic collaborations. These include the ability to store energy; efficient light-driven chemistry, materials, and devices; low-energy methods to change materials like nitrogen and carbon dioxide to useful chemicals and fuels; and optimal use of natural gas. The research priorities will continue to evolve through the exchange and cross-fertilization that the Vagelos Institute fosters.
Teams of researchers also formed to create proposals for the first Vagelos Institute seed grants. “You can apply for an outside grant together, but it’s hard to be successful with no history of collaboration,” says Goldberg. “This is allowing people to get together, start a project, and then as a team apply for federal money with good evidence of the potential of the project.” The first set of projects ranges from finding ways to recycle carbon dioxide to make fuels and useful chemicals, to using light to catalyze energy transformations, to creating completely new materials that could allow sodium-ion batteries to replace batteries made with the much rarer lithium.
“We’re seeing faculty who haven’t been involved in specific fields of research who are getting the opportunity to try new things,” says Nadine Gruhn, Vagelos Institute Managing Director and another CENTC veteran. In one project, researchers are combining different methods of catalysis to see if they can get the best of both types, with computational scientists also adding their expertise.
The Institute complements other energy-related initiatives at Penn, including the Kleinman Center for Energy Policy and the Penn Program for Environmental Humanities, which seeks a deeper understanding of how humans interact with the natural world. They also interact with the Penn Center for Innovation, and Goldberg cites the University’s connections to industry as another strength in their mission: “That has to be a piece of the puzzle. You have to be thinking about solutions that are going to work on a huge industrial scale, and it’s good to know early on if a strategy is going to be able to translate.”
Not only is the Vagelos Institute at the fore in bringing top researchers together for collaborative energy research and solutions, it is also training the next generation of energy leaders through graduate and postdoctoral fellowships. The fellows are included in the regular Institute meetings and are involved in all stages of the discussion and research.
The researchers brought together by the Vagelos Institute have expertise in a range of disciplines, including chemical and biomolecular engineering, mechanical engineering, physics, and earth and environmental science. A critical mass of participating faculty are from the Department of Chemistry, with specialties across biological, inorganic, organic, and physical chemistry.
“Everyone has a different perspective, a different approach to science, even people who are in the same field,” says Goldberg. “There’s no one way and no one answer, but the solutions will start from here.”
A New Home for Energy Science and Technology
Energy research at Penn got a boost from a recent gift from P. Roy Vagelos, C’50, PAR’90, HON’99, and Diana T. Vagelos, PAR’90 (p. 22), to be used for a new, state-of-the-art science center to house researchers focused on energy science and technology.
“The Vageloses are putting Penn’s energy research effort into superdrive with their very generous gift for a new building,” says Karen Goldberg, Vagelos Professor in Energy Research in the Department of Chemistry and Vagelos Institute Director.
“Energy research is highly interdisciplinary in nature, drawing from all the major areas of chemistry—biological, inorganic, organic, and physical chemistry, as well as engineering and other sciences,” says David Christianson, Roy and Diana Vagelos Professor in Chemistry and Chemical Biology and Chair of the Department of Chemistry. “The enduring legacy of this gift will fuel decades of discovery in the molecular sciences at Penn.”
Chemists Take on the Energy Challenge
From human-made photosynthesis to transforming “waste” products into useful materials to developing better plastics, here are some of the perspectives that chemists in Penn Arts & Sciences bring to bear as we find our way to sustainable solutions.
CATALYZING CHANGE
Karen Goldberg, Vagelos Professor in Energy Research
Methane gas (CH4) and methanol (CH3OH) are differentiated by just one oxygen atom, but to make the first into the second requires a billion-dollar facility and temperatures between 400 and 600 degrees Fahrenheit.
“All you’ve done is insert an oxygen. But the commercial process to do that takes the methane, breaks every one of the C-H bonds to make carbon monoxide, and then puts three of the C-H bonds back again,” says Karen Goldberg, Vagelos Professor in Energy Research. “This process was developed in the early 20th century. It was fantastic for its time, but overall, it’s very energy intensive and fundamentally inefficient. Today, we know a lot more about how to promote the transformation of different types of bonds. For example, we now know how to use a metal to selectively cleave one C-H bond and leave the other C-H bonds untouched. So we’re working hard to figure out how we can insert an oxygen atom selectively and make methanol by a more direct and efficient process.”
Goldberg’s research focuses on the development of new catalytic systems to efficiently produce chemicals and fuels from a range of available feedstocks, such as natural gas and carbon dioxide. By developing a detailed understanding of fundamental reactions, her lab seeks to develop new environmentally responsible and economically viable methods.
“What kept me going on to graduate school after college was the idea that you could go to work every day and learn something new,” says Goldberg, who is the Director of the Vagelos Institute for Energy Science and Technology. “And not only that, you could learn things that nobody else yet knew.”
CHEMISTRY AT THE NANOSCALE
Thomas Mallouk, Vagelos Professor in Energy Research
Nanomaterials research has enabled the creation of innovative products ranging from mechanical lubricants to super-light carbon bicycle frames. But while materials made at the nanoscale are by definition small, the challenges surrounding the design and creation of these strong, resilient, and energy-efficient materials are anything but miniscule.
Researchers in the lab of Thomas Mallouk, the second Vagelos Professor in Energy Research, work on nanoscale chemistry and assembly techniques that can be used to make nanomaterials with unique properties that can carry out specific tasks. While Mallouk started his career studying how chemical bonds form, he quickly found his niche in the research community by combining ideas from the molecular world with solid-state physics and engineering.
Mallouk and his group tackle a broad range of topics in materials science by incorporating techniques from synthetic chemistry. “A lot of our work is discovery-based,” Mallouk says of his group. “We think about how we can use what we understand well in order to solve other people’s problems.” His team has worked across a range of applications, from conductive materials that are only a few atoms thick to systems that can mimic the light-capturing abilities of photosynthesis.
The group’s work has led them toward even more diverse applications, including nanoscale electronics and new methods for making catalysts. He also has experience working with fuel cells, chemically powered micro robots, and materials that convert chemical energy into mechanical energy. His group was part of a project with civil engineering researchers that used nanomaterials to clean up toxic substances in ground water.
MAKING THE MOST OUT OF METHANE
Daniel J. Mindiola, Brush Family Professor of Chemistry
Methane makes up more than 80 percent of all natural gas resources. But while methane is energy-rich, incredibly abundant, and convenient to extract from rock and oil reservoirs, it is a difficult molecule to store, transport, and use as a chemical reagent. This natural resource is approximately 25 times more potent a greenhouse gas than carbon dioxide. Due to its strong carbon-hydrogen bonds, working with methane also requires extreme conditions, such as very high temperatures or pressures, so nearly 15 percent of the total methane extracted worldwide is simply burned away in massive flare stacks that can be as large as an entire city.
Daniel J. Mindiola, Brush Family Professor of Chemistry, and his research group are currently working on how to use methane to create more value-added carbon-based materials that are easier to work with and store than methane gas. They want to use methane as a building block for making more complex chemicals that have more value in industry, such as plastics, polymers, solvents, detergents, adhesives, and other household products. “There’s a lot of energy stored in those bonds,” he says. “Rather than burning the methane, let’s think of something more environmentally friendly to do with this vast resource and where we can still retain that chemical energy.”
His group uses fundamental molecular-level chemistry to study specific reactions involving the conversion of methane so they can better understand the mechanisms and pathways involved in the transformations of this molecule. One of their goals is to identify reaction pathways involving methane transformations that can be carried out under mild conditions while using catalysts that are abundant, cheap, and sustainable. “If you know how the system works, you can improve it. If you know what the bottleneck of that reaction is, or what is slowing down that reaction, you can look at conditions that can improve the reaction’s efficiency,” says Mindiola.
SHINING A LIGHT ON CERIUM
Eric Schelter, Professor of Chemistry
Chemists rely on rare metals like platinum, ruthenium, and iridium to drive certain chemical reactions, but extracting these difficult-to-find metals requires a substantial amount of time and energy. One example is neodymium, a metal that is used to make the permanent magnets for wind turbines. When neodymium is mined, the extracts contain high amounts of a second metal known as cerium, which is considered a “waste” product of neodymium mining.
Researchers in the lab of Eric Schelter, Professor of Chemistry, are taking advantage of this “waste” element’s unique ability to both absorb and emit light. This work started when one of Schelter’s students was making a complex containing cerium in the lab and was surprised when the chemical solution started to glow. His group is now studying the chemical structure of this and related cerium systems to understand how they capture light energy. “What happens with this cerium complex is very different from anywhere else on the periodic table. This element has a huge potential to drive a lot of interesting energy-related chemistry,” Schelter says.
His goal is to develop cerium-containing catalysts that can efficiently absorb light and activate small molecules, such as components of natural gas, into more complex carbon molecules. “If you want to really transform chemical fuels, you have to do that chemistry with elements that are abundant, like cerium,” he says.
SUSTAINABLE MATERIALS
Zahra Fakhraai, Associate Professor of Chemistry
Even if carbon generation were to end entirely, there would still be a significant amount of carbon that would need to be captured; if all plastic production were halted, there would still be an enormous amount of polymer waste that would need to be cleaned up. Advances in fundamental chemistry are crucial for developing new technologies that can solve these problems.
Research done in the lab of Zahra Fakhraai, Associate Professor of Chemistry, is focused on creating sustainable and innovative materials through fundamental materials science research. She works on two different energy-related problems. One of her projects includes the use of optics to figure out how light can be manipulated at incredibly small scales in order to harvest and transfer light energy. She also does fundamental research on how polymers behave on the nanoscale, which will allow materials scientists to create plastic products that are more durable and reusable and that enables polymers to be recycled more efficiently.
While Fakhraai works on the fundamental aspects of materials science, she also enjoys working on problems with semi-immediate applications that help motivate the questions that her group will address. “What’s lagging behind in solving these environmental problems are materials and chemistries that could mitigate the effects of climate change and plastic pollution,” says Fakhraai. “The technologies here are very limited, and we need research at the basic level to understand how you would do these things.”
COMPUTATIONAL DESIGN
Andrew M. Rappe, Blanchard Professor of Chemistry
Materials scientists and engineers want to design materials that harness, convert, and use energy more efficiently and can also be easily reused. However, there are an infinite number of chemical combinations and a finite amount of resources available to try out new combinations. By using an approach known as computational materials design, researchers can specify what they need a material to do and can use a model that allows them to identify and design new materials more efficiently.
Andrew M. Rappe, Blanchard Professor of Chemistry, and his group use computational approaches to study systems in materials science, condensed-matter physics, and physical chemistry. His research provides a glimpse into what solids are doing at the atomic level and allows researchers to predict how new materials will work even if they’ve never been made before. Rappe and his group work alongside engineers and experimental chemists to help validate their predictions while also helping their colleagues understand the results they find. “This loop between theory and experiment has enabled rapid progress within the alternative energy area,” says Rappe.
Rappe is also the Co-Director of the Vagelos Integrated Program in Energy Research (VIPER), which offers undergraduate students dual degrees in science and engineering and provides opportunities for research and mentorship.
CAPTURING ENERGY FROM LIGHT
Jessica M. Anna, Assistant Professor of Chemistry and Elliman Faculty Fellow
Photosynthesis is an essential and fundamental chemical process used by plants to convert sunlight into energy. The very first step of photosynthesis, which involves capturing solar energy, is extremely efficient: For every photon of light a plant absorbs, it captures the light’s energy and converts nearly all of it into a charge-separated state that drives photosynthesis. But while scientists have studied photosynthesis for many years, there are still uncertainties about how this process works and how it became so energy-efficient.
Jessica M. Anna, Assistant Professor of Chemistry and Elliman Faculty Fellow, is interested in understanding energy and electron transfer processes using systems that are relevant to solar energy conversion. Her group studies both artificial light-harvesting systems and photosynthetic complexes that are harvested from plants. What Anna finds intriguing is that whether her group is studying a molecule with only 18 atoms or a complex photosynthesis structure that contains hundreds of molecules of chlorophyll, they all capture light energy with incredible efficiency. “We’re really trying to understand the molecular-level parameters that lead to this energy-level landscape,” says Anna.
Anna’s group specializes in using multi-dimensional spectroscopy to capture detailed glimpses into the dynamics and structure of light-harvesting complexes. She is now using her custom spectrometer to bring together both visible light and infrared techniques in order to more closely study systems that are relevant for solar energy conversion.
For Anna, a fundamental approach to chemistry research is crucial for progress in the area of alternative energy. “Once you understand these reactions at a fundamental level, you can start to engineer these principles into light-harvesting materials and design materials which are inspired by systems in biology,” she says.
SYNTHESIZING CHEMICALS SUSTAINABLY
Marisa Kozlowski, Professor of Chemistry
Organic chemicals, or chemical compounds that contain carbon, include a broad range of materials such as pharmaceuticals, fragrances, food additives, cosmetics, and agricultural chemicals. However, the process of manufacturing many of these organic chemicals on an industrial scale uses a substantial amount of energy. Chemists want to create these chemicals more sustainably, but in order to do so need to have a solid understanding of the mechanisms involved with each step in their synthesis.
Marisa Kozlowski, Professor of Chemistry, is focused on developing new, highly efficient methods for creating complex organic chemicals. Her group is looking for ways to convert biomass, or waste materials from plants, and small hydrocarbons, such as carbon dioxide, into chemicals that can be used in industrial-scale synthesis. Kozlowski’s group is focused on reactions that use oxygen and common metals, such as iron and nickel, instead of relying on conventional approaches that require rare, expensive, or toxic metals.
Kozlowski’s group uses a systematic approach to decide what reactions they want to study by looking at what areas are missing or have inefficient reactions. Her group has made some surprising discoveries that demonstrate an unexpected level of control for certain reactions. “We discovered a process where we could do this reaction with extraordinarily high efficiency that we didn’t understand until recently. Thanks to that finding, the field is limitless.”